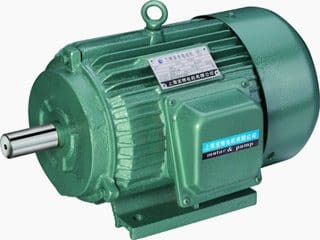
This simplest form of AC induction motor or asynchronous motor is the basic, universal workhorse of industry. Its general construction is shown in Fig. 1. It is usually designed for fixed-speed operation, larger ratings having such features as deep rotor bars to limit Direct on Line (DOL) starting currents.
Electronic variable speed drive technology is able to provide the necessary variable voltage, current and frequency that the induction motor requires for efficient, dynamic and stable variable speed control.
Modern electronic control technology is able not only to render the AC induction motor satisfactory for many modern drive applications but also to extend greatly its application and enable users to take advantage of its low capital and maintenance costs.
More striking still, microelectronic developments have made possible the highly dynamic operation of induction motors by the application of flux vector control. The practical effect is that it is now possible to drive an AC induction motor in such a way as to obtain a dynamic performance in all respects better than could be obtained with a phase-controlled dc drive combination.
The stator winding of the standard industrial induction motor in the integral kilowatt range is three-phase and is sinusoidally distributed. With a symmetrical three-phase supply connected to these windings, the resulting currents set up, in the air-gap between the stator and the rotor, a travelling wave magnetic field of constant magnitude and moving at synchronous speed. The rotational speed of this field is f/p revolutions per second, where f is the supply frequency (hertz) and p is the number of pole pairs (a four-pole motor, for instance, having two pole pairs). It is more usual to express speed in revolutions per minute, as 60 f/p (rpm).
The emf generated in a rotor conductor is at a maximum in the region of maximum flux density and the emf generated in each single rotor conductor produces a current, the consequence being a force exerted on the rotor which tends to turn it in the direction of the flux rotation. The higher the speed of the rotor, the lower the speed of the rotating stator flux field relative to the rotor winding, and therefore the smaller is the emf and the current generated in the rotor cage or winding.
The speed when the rotor turns at the same rate as that of the rotating field is known as synchronous speed and the rotor conductors are then stationary in relation to the rotating flux. This produces no emf and no rotor current and therefore no torque on the rotor. Because of friction and windage the rotor cannot continue to rotate at synchronous speed; the speed must therefore fall and as it does so, rotor emf and current, and therefore torque, will increase until it matches that required by the losses and
by any load on the motor shaft. The difference in rotor speed relative to that of the rotating stator flux is known as the slip.
by any load on the motor shaft. The difference in rotor speed relative to that of the rotating stator flux is known as the slip.
It is usual to express slip as a percentage of the synchronous speed. Slip is closely proportional to torque from zero to full load.

The most popular squirrel cage induction motor is of a 4-pole design. Its synchronous speed with a 50 Hz supply is therefore 60 f/p, or 1500 rpm. For a full-load operating slip of 3 per cent, the speed will then be (1 – s)60 f/p, or 1455 rpm.
Torque characteristics
A disadvantage of the squirrel cage machine is its fixed rotor characteristic. The starting torque is directly related to the rotor circuit impedance, as is the percentage slip when running at load and speed. Ideally, a relatively high rotor impedance is required for good starting performance (torque against current) and a low rotor impedance provides low full-load speed slip and high efficiency.

This problem can be overcome to a useful extent for DOL application by designing the rotor bars with special cross sections as shown in Fig. 2 so that rotor eddy currents increase the impedance at starting when the rotor flux (slip) frequency is high.
Alternatively, for special high starting torque motors, two or even three concentric sets of rotor bars are used. Relatively costly in construction but capable of a substantial improvement in starting performance, this form of design produces an increase in full load slip. Since machine losses are closely proportional to working speed slip, increased losses may require such a high starting torque machine to be derated.

The curves in Fig. 3 indicate squirrel cage motor characteristics. In the general case, the higher the starting torque the greater the full load slip. This is one of the important parameters of squirrel cage design as it influences the operating efficiency.
No comments:
Post a Comment